Issue
How does our immune system react to a viral infection?
Our immune system has evolved as a multi-component entity that can be seen as having 2 lines of defence: innate immunity and adaptive immunity. Although the innate and adaptive immune systems are closely linked in many ways, they are each made up of different cell types with different functions.
In an example of a general viral infection, innate immunity represents the first line of defence. It ‘sounds the alarm’ immediately or within hours of encountering an antigen, slows down the replication and spread of the virus, and triggers the adaptive immune response to the intruding pathogen. Adaptive immunity is antigen-dependent and antigen-specific, and therefore involves a delay between exposure to the antigen and the maximum response (6-10 days). It is made up of 3 main types of cells: antibody-producing B cells, CD4+ T cells with a range of helper and effector functions, and CD8+ T cells that kill infected cells.
In simple terms, antibodies mainly eliminate viruses outside cells, while T cells eliminate viruses inside cells. This powerful division of labour has been shown to be an effective complementary approach to controlling most viral infections in most individuals [2].
The SARS-CoV-2 virus is particularly effective at avoiding or delaying the onset of intracellular immune responses. As a result, the virus replicates relentlessly and adaptive immune responses are not initiated until the innate immune alarm is triggered [3,4]. Patients suffering from Covid-19 present varying degrees of severity in terms of symptoms.
Approximately 40% of SARS-CoV-2 infections are asymptomatic or mild because T-cell and antibody responses occur relatively rapidly and control the infection. Indeed, the presence of T cells and antibodies is associated with recovery in most of these Covid-19 cases [5]. On the other hand, if the delay in the innate immune response is too long, the virus takes a considerable lead in its replication in the upper respiratory tract and lungs, and the adaptive immune response is not initiated, leading to a severe form of lung disease and hospitalisation [6].
Description
Does immunity to SARS-CoV-2 already exist?
SARS-CoV-2 belongs to the family of coronaviruses; RNA viruses that infect numerous animal species. Six other coronaviruses are known to be associated with respiratory tract infections in humans. Four of these, all common human coronaviruses (HCoV), are endemically transmitted and known to cause the common cold (229E, NL63, OC43, HKU1), while SARS-CoV and Middle East Respiratory Syndrome Coronavirus (MERS-CoV) cause severe forms of endemic pneumonia [7]. Given that many people have been exposed to colds caused by HCoV, it is of interest to investigate whether there is pre-existing immunity to SARS-CoV-2, also known as cross-immunity, due to previous exposure to endemic HCoV [8,9].
Several studies have described the prevalence of cross-reactive T cells against SARS-CoV-2 in subjects never exposed to SARS-CoV-2 [5,10-12] and in patients hospitalised with Covid-19 [13,14]. The majority of T cells cross-reacting against SARS-CoV-2 were CD4+ T cells [5], characterised as memory T cells, and many were memory T cells for common cold HCoV with epitopes conserved between these viruses [10,15]. Cross-reacting CD8+ T cells have been observed less frequently [5], but may have an appropriate biological role [16]. The presence of cross-reactive immunity in a proportion of the population is of great interest, as it opens the door to a certain level of pre-existing immunity in the population [17]. For example, in the case of the 2009 H1N1 pandemic, there was an unusual distribution of disease severity, with older people faring better than younger adults. This correlated with the circulation of a different strain of H1N1 in the human population decades earlier, which generated pre-existing immunity in people old enough to have been exposed to it [18,19]. Epidemiological evidence from a large cohort study now supports the possibility of some degree of pre-existing immunity: people infected with SARS-CoV-2 with confirmed HCoV infection in the previous 3 years had a significantly lower risk of ICU admission, after controlling for age and other factors [20].
Results
Future prospects
There is increasing evidence that pre-existing immune reactivity to SARS-CoV-2 exists to some degree in the general population. However, researchers have reported inconsistent results in measuring cross-reactivity between SARS-CoV-2 and common cold HCoV, due to differences in research subjects (of different ages, sexes, and geographical origins), tests, and test cut-off values. The tests have not yet been standardised and may vary from one laboratory to another in terms of target antigens, products used, protocols, and interpretations. Prospective, carefully designed, larger-scale studies evaluating the clinical outcomes of SARS-CoV-2 infection in SARS-CoV-2-unexposed individuals with cross-reactive T cells will be required to provide conclusive evidence regarding the protective potential of these cross-reactive T cells.
References
1. WHO WHO Coronavirus (COVID-19) Dashboard | WHO Coronavirus (COVID-19) Dashboard With Vaccination Data Available online: https://covid19.who.int/ (accessed on Feb 8, 2022).
2. Weaver, C.; Murphy, K. Janeway’s Immunobiology; Norton, W.W., Ed.; 2016;
3. Arunachalam, P.S.; Wimmers, F.; Mok, C.K.P.; Perera, R.A.P.M.; Scott, M.; Hagan, T.; Sigal, N.; Feng, Y.; Bristow, L.; Tsang, O.T.Y.; et al. Systems biological assessment of immunity to mild versus severe COVID-19 infection in humans. Science 2020, 369, 1210–1220, doi:10.1126/SCIENCE.ABC6261.
4. Sette, A.; Crotty, S. Adaptive immunity to SARS-CoV-2 and COVID-19. Cell 2021, 184, 861–880, doi:10.1016/j.cell.2021.01.007.
5. Grifoni, A.; Weiskopf, D.; Ramirez, S.I.; Mateus, J.; Dan, J.M.; Moderbacher, C.R.; Rawlings, S.A.; Sutherland, A.; Premkumar, L.; Jadi, R.S.; et al. Targets of T Cell Responses to SARS-CoV-2 Coronavirus in Humans with COVID-19 Disease and Unexposed Individuals. Cell 2020, 181, 1489–1501.e15, doi:10.1016/j.cell.2020.05.015.
6. Rydyznski Moderbacher, C.; Ramirez, S.I.; Dan, J.M.; Grifoni, A.; Hastie, K.M.; Weiskopf, D.; Belanger, S.; Abbott, R.K.; Kim, C.; Choi, J.; et al. Antigen-Specific Adaptive Immunity to SARS-CoV-2 in Acute COVID-19 and Associations with Age and Disease Severity. Cell 2020, 183, 996–1012.e19, doi:10.1016/J.CELL.2020.09.038.
7. Cui, J.; Li, F.; Shi, Z.L. Origin and evolution of pathogenic coronaviruses. Nat. Rev. Microbiol. 2019, 17, 181–192, doi:10.1038/s41579–018–0118–9.
8. Khanolkar, A. Elucidating T cell and B cell responses to SARS-CoV-2 in humans: Gaining insights into protective immunity and immunopathology. Cells 2022, 11, doi:10.3390/cells11010067.
9. Shrwani, K.; Sharma, R.; Krishnan, M.; Jones, T.; Mayora-Neto, M.; Cantoni, D.; Temperton, N.J.; Dobson, S.L.; Subramaniam, K.; McNamara, P.S.; et al. Detection of Serum Cross-Reactive Antibodies and Memory Response to SARS-CoV-2 in Prepandemic and Post-COVID-19 Convalescent Samples. J. Infect. Dis. 2021, 224, 1305–1315, doi:10.1093/infdis/jiab333.
10. Mateus, J.; Grifoni, A.; Tarke, A.; Sidney, J.; Ramirez, S.I.; Dan, J.M.; Burger, Z.C.; Rawlings, S.A.; Smith, D.M.; Phillips, E.; et al. Selective and cross-reactive SARS-CoV-2 T cell epitopes in unexposed humans. Science (80-. ). 2020, 370, 89–94, doi:10.1126/science.abd3871.
11. Nelde, A.; Bilich, T.; Heitmann, J.S.; Maringer, Y.; Salih, H.R.; Roerden, M.; Lübke, M.; Bauer, J.; Rieth, J.; Wacker, M.; et al. SARS-CoV-2-derived peptides define heterologous and COVID-19-induced T cell recognition. Nat. Immunol. 2021, 22, 74–85, doi:10.1038/s41590–020–00808-x.
12. Le Bert, N.; Tan, A.T.; Kunasegaran, K.; Tham, C.Y.L.; Hafezi, M.; Chia, A.; Chng, M.H.Y.; Lin, M.; Tan, N.; Linster, M.; et al. SARS-CoV-2-specific T cell immunity in cases of COVID-19 and SARS, and uninfected controls. Nature 2020, 584, 457–462, doi:10.1038/s41586–020–2550-z.
13. Ferretti, A.P.; Kula, T.; Wang, Y.; Nguyen, D.M.V.; Weinheimer, A.; Dunlap, G.S.; Xu, Q.; Nabilsi, N.; Perullo, C.R.; Cristofaro, A.W.; et al. Unbiased Screens Show CD8+ T Cells of COVID-19 Patients Recognize Shared Epitopes in SARS-CoV-2 that Largely Reside outside the Spike Protein. Immunity 2020, 53, 1095–1107.e3, doi:10.1016/j.immuni.2020.10.006.
14. Bacher, P.; Rosati, E.; Esser, D.; Martini, G.R.; Saggau, C.; Schiminsky, E.; Dargvainiene, J.; Schröder, I.; Wieters, I.; Khodamoradi, Y.; et al. Low-Avidity CD4+ T Cell Responses to SARS-CoV-2 in Unexposed Individuals and Humans with Severe COVID-19. Immunity 2020, 53, 1258–1271.e5, doi:10.1016/j.immuni.2020.11.016.
15. Kundu, R.; Narean, J.S.; Wang, L.; Fenn, J.; Pillay, T.; Fernandez, N.D.; Conibear, E.; Koycheva, A.; Davies, M.; Tolosa-Wright, M.; et al. Cross-reactive memory T cells associate with protection against SARS-CoV-2 infection in COVID-19 contacts. Nat. Commun. 2022, 13, 1–8, doi:10.1038/s41467–021–27674-x.
16. Schulien, I.; Kemming, J.; Oberhardt, V.; Wild, K.; Seidel, L.M.; Killmer, S.; Sagar; Daul, F.; Salvat Lago, M.; Decker, A.; et al. Characterization of pre-existing and induced SARS-CoV-2-specific CD8+ T cells. Nat. Med. 2021, 27, 78–85, doi:10.1038/s41591–020–01143–2.
17. Sette, A.; Crotty, S. Pre-existing immunity to SARS-CoV-2: the knowns and unknowns. Nat. Rev. Immunol. 2020, 20, 457–458, doi:10.1038/s41577–020–0389-z.
18. Greenbaum, J.A.; Kotturi, M.F.; Kim, Y.; Oseroff, C.; Vaughan, K.; Salimi, N.; Vita, R.; Ponomarenko, J.; Scheuermann, R.H.; Sette, A.; et al. Pre-existing immunity against swine-origin H1N1 influenza viruses in the general human population. Proc. Natl. Acad. Sci. U. S. A. 2009, 106, 20365–20370, doi:10.1073/pnas.0911580106.
19. Hancock, K.; Veguilla, V.; Lu, X.; Zhong, W.; Butler, E.N.; Sun, H.; Liu, F.; Dong, L.; DeVos, J.R.; Gargiullo, P.M.; et al. Cross-Reactive Antibody Responses to the 2009 Pandemic H1N1 Influenza Virus. N. Engl. J. Med. 2009, 361, 1945–1952, doi:10.1056/nejmoa0906453.
20. Sagar, M.; Reifler, K.; Rossi, M.; Miller, N.S.; Sinha, P.; White, L.F.; Mizgerd, J.P. Recent endemic coronavirus infection is associated with less-severe COVID-19. J. Clin. Invest. 2021, 131, doi:10.1172/JCI143380.
Contact
us
Discover also
Tailored solutions for your needs
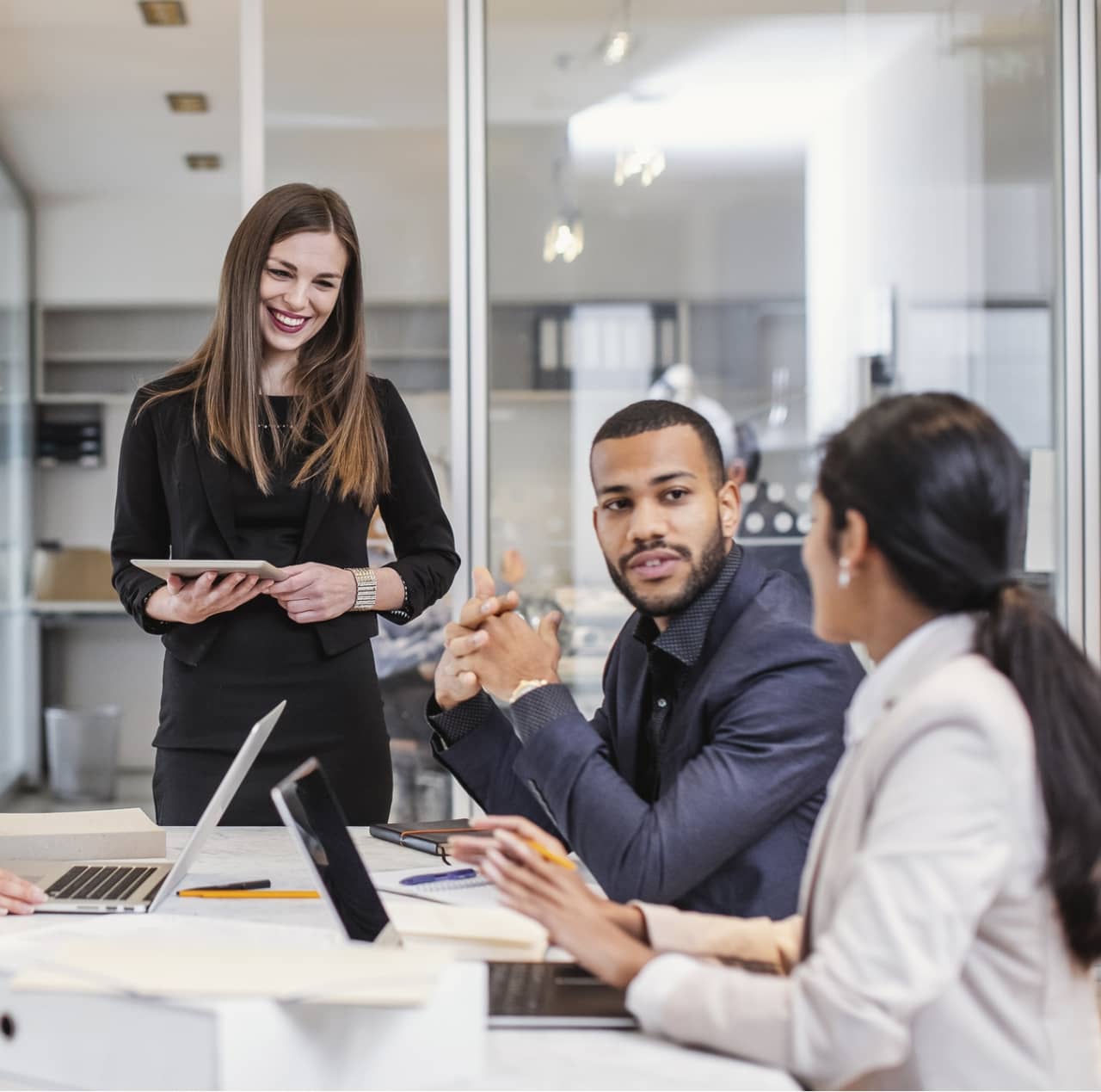
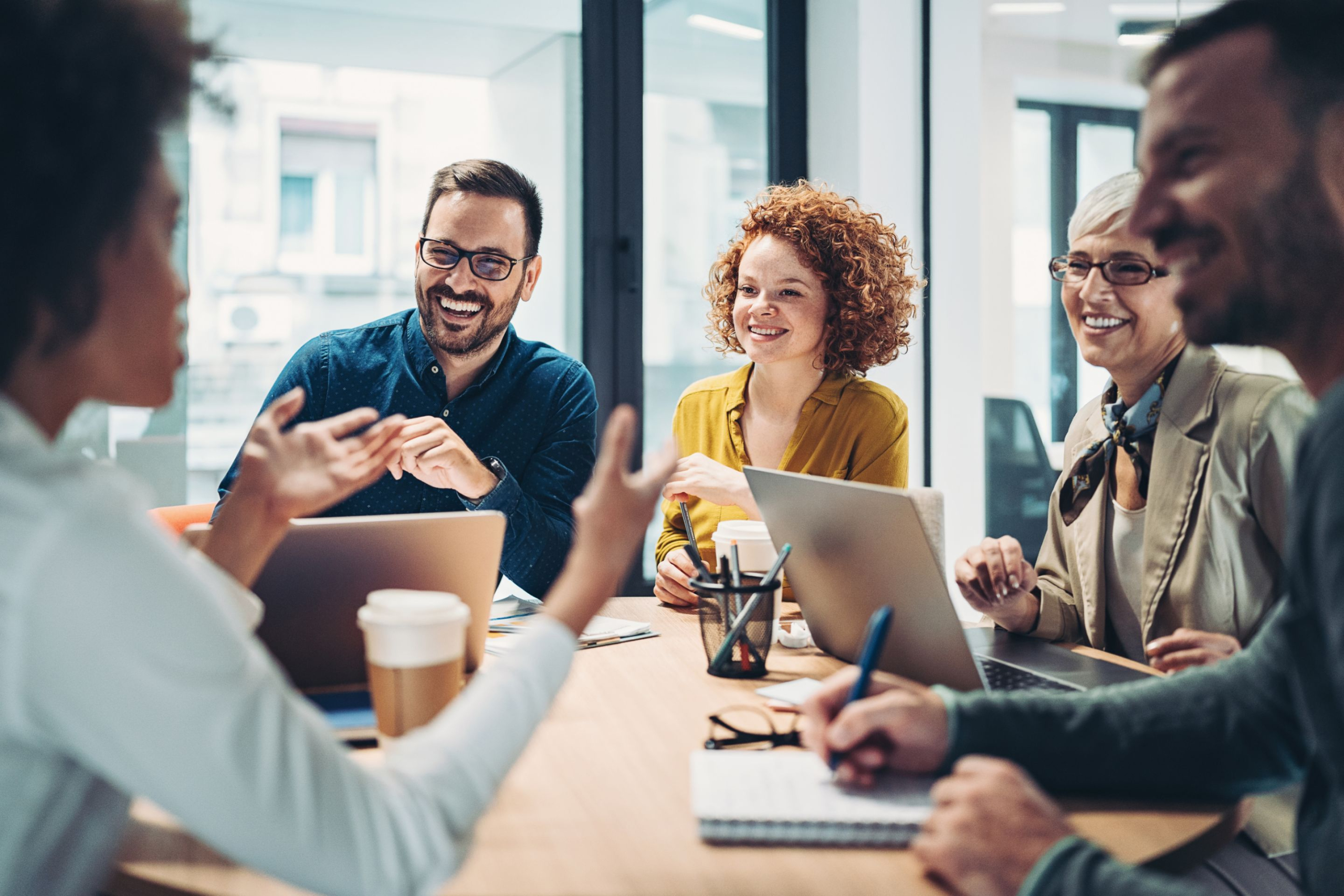
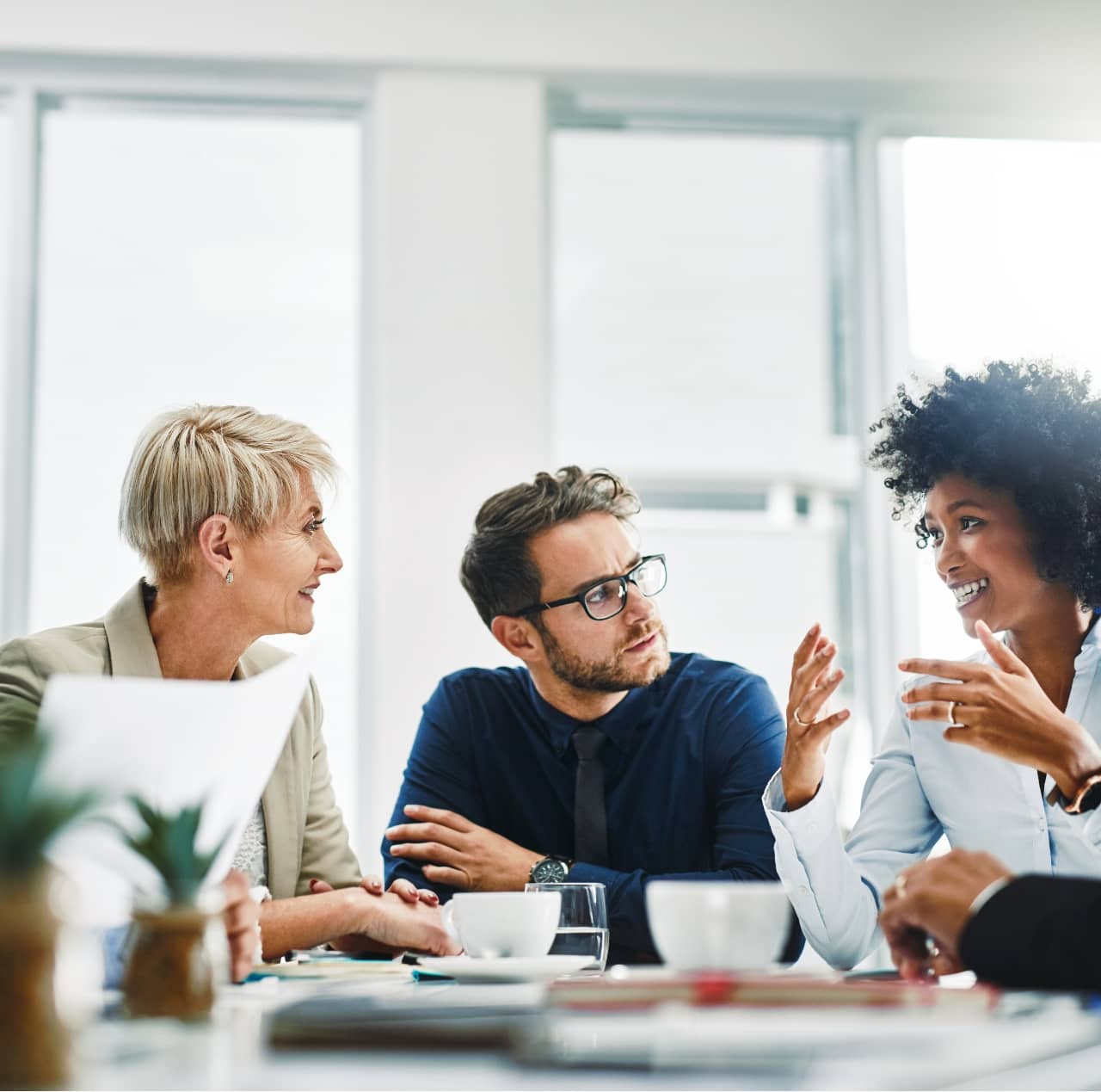